Welcome to the fourth of six articles in our analysis and compliance in pharmaceuticals series. In this post we explore ion chromatography and its versatile methods for drug identification in the pharmaceutical industry.
Ion chromatography is a flexible technique with a wide variety of practical uses in the pharma sector. Here we take a look at some pertinent trends and recent advances in its application.
Ion Chromatography Testing in a Regulated Environment
High standards have to be met by the pharmaceutical industry when it comes to drug quality and safety. These standards are documented in pharmacopoeias as officially recognized pharmaceutical rules and published as legal tools of customer protection by authorities such as governments and medical societies. The identification of a drug depends on sensitive, reliable instruments, and methods – as does the determination of the drug’s compliance with applicable regulations.
Ion chromatography (IC) is the method of choice to determine active ingredients, excipients, and traces of impurities, as well as metabolites in the form of organic and inorganic ions or polar substances, in a number of pharmaceuticals, pharmaceutical solutions, and even body fluids. It can determine the presence of several substances within a very short time in a single analysis and even distinguish chemically similar analytes. The concentration of analytes can vary from ng/L up to the percent range. The large selection of separation columns and elution systems available makes IC useful for almost any kind of analyte.
Interfering effects caused by the sample matrix can easily be avoided by using the right sample preparation or choosing a suitable detection method. Inline sample preparation is a feature of many modern IC systems, as the focus of recent advances in IC has been mainly on ease of use. However, convenience is not the only advantage brought by the automation of the IC process; reducing human interference to a minimum also means reducing the chances of mistakes and contamination. Depending on the requirements of the analyte and matrix, a broad range of detection methods is available:
- Conductivity detection with and without suppression
- Electrochemical detection
- Spectrophotometric detection with and without post-column derivatisation (ultraviolet–visible spectrophotometry)
- Coupled detection methods such as IC–mass spectrometry (MS) and IC–inductively-coupled-plasmaMS
Pharmaceutical samples come in many different forms which require different ion chromatographic approaches. What follows is an overview of frequent sample types with relevant example analyses.
Pharmaceutical Solutions
The term ‘pharmaceutical solutions’ denotes isotonic, hemodialysis, or infusion solutions. They contain anions, cations, carbohydrates, and organic acids, the concentrations of which frequently differ from one another by several orders of magnitude. Within the context of production monitoring and final quality control, an analysis method is required that can determine these ingredients with a high degree of precision. In addition, the analysis should be quick and require minimal effort. With its intelligent analytical procedure and automatic inline sample preparation, IC fully accomplishes this task.
Two example analyses of hemodialysis solutions are shown in Figures 1 – 2. Patients suffering from renal failure require hemodialysis to compensate for the loss of the kidney’s blood cleansing function. During the process, the patient’s blood exchanges solutes with a hemodialysis solution through a semi-permeable membrane. The exchanged solutes include, among others, waste products such as urea and phosphate, which diffuse out of the blood and into the dialysis solution along the concentration gradient.
The composition of dialysis solutions is complex, because the removal of solutes from the blood changes its osmotic activity; therefore, it has to take place at a controlled rate, which is achieved by the right solute concentration. A strong change in osmotic activity can cause dialysis disequilibrium syndrome where, due to the low solute concentration in blood, solutes are washed out from other body compartments.
Figure 1 shows the simultaneous determination of citrate and acetate in diluted hemodialysis solution. In part A, an anion standard was measured; part B shows the sample determination. Citrate is added to hemodialysis solutions for its anticoagulant properties and acetate is added as a buffer substance. It is transferred to the patient’s bloodstream during hemodialysis and stabilizes the blood’s pH value. This is necessary because the kidneys of dialysis patients are not capable of excreting acid components – therefore, patients are often acidotic.
Besides citrate and acetate, the chromatogram reveals the presence of a nearly physiological concentration of chloride. By using physiological solute concentrations, the concentration gradient is reduced to a minimum, and a dynamic equilibrium is reached between blood and dialysis solution. The loss of certain solutes – including chloride – is thereby prevented. Figure 2 shows the determination of cations in hemodialysis concentrate after an automated inline dilution step. Like chloride, the cations are present in nearly physiological concentrations to avoid their drainage from patients’ blood by osmosis.
Active Pharmaceutical Ingredients
Active pharmaceutical ingredients (APIs) in medicines such as gentamicin, neomycin, cefadroxil, or bethanechol chloride can be determined by IC in accordance with the regulations of the US Pharmacopeia (USP) and the European Pharmacopoeia. The requirements regarding precision, separation, and recovery of the analytes are described in detail in the pharmacopoeias. Figure 3 depicts the ion chromatogram of an analysis of gentamicin, an antibiotic belonging to the group of aminoglycosides. Aminoglycosides are bactericidal antibiotics that block bacterial protein biosynthesis by binding to ribosomes, thereby causing mistakes in the translation from messenger ribonucleic acid to DNA. Gentamicin consists of several closely related compounds, namely gentamicin C1, gentamicin C1a, and gentamicin C2, C2a, and C2b. In spite of their structural similarity, IC achieves a good separation of the different gentamicin components.
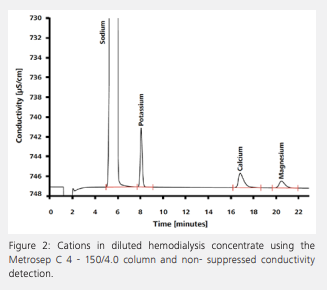
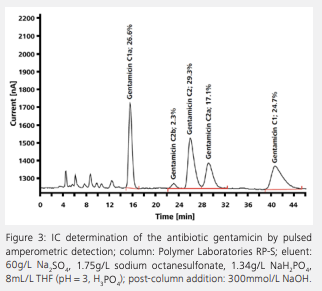
Impurities in Pharmaceuticals
Impurities in pharmaceutical products can also be determined by IC. Even small concentrations of an impurity can cause significant side effects. For example, in the synthesis of the antihypertensive irbesartan, azide can be detected in traces as an impurity in the product. Azide is strongly toxic to humans and its concentration in irbesartan is therefore subject to rigorous controls. According to USP 621, the US Pharmacopeia recommends ion chromatographic azide determination after direct injection. In this method, a transfer solution consisting of the IC eluent and suitable organic solvent is used to remove the API from the analytical column. However, this procedure is tedious, time consuming, and cannot be automated. Azide determination is more selective, more sensitive, and, above all, quicker with the use of inline matrix elimination. The interfering pharmaceutical matrix is separated from the target analyte in the course of sample preparation. The ion chromatogram shows the analysis of an irbesartan sample spiked with different concentrations of azide (See Figure 4).
High Sensitivity Thanks to Matrix Elimination
The signal is recorded by a conductivity detector following sequential suppression. Table 1 lists the average recovery values of azide that were achieved over three measurements, the mean conductivity measured by the detector, and the relative standard deviation. The determination of azide in irbesartan with preceding matrix elimination fulfills all requirements of the regulatory authorities, including the selectivity of the method, its limits of detection and quantitation, precision, linearity, accuracy, and robustness. Thus, it can be used as a quicker and more sensitive alternative to the proposed determination according to USP 621.
Table 1. Precision and Recovery of Azide | |||
Peak Area | |||
Mean value (μS/cm) | Relative Standard Deviation (%) | Recovery (%) | |
5 µg/L spike | ± 5.00 | 1.96 | 101.71 |
30 µg/L spike | ± 0.30 | 0.14 | 103.38 |
Radio Ion Chromatography
Radio IC aims to determine the radiochemical purity of radiopharmaceuticals. These are radioactive substances that are used for medical purposes, mainly in diagnostics, but also in the treatment and prevention of certain diseases. [18F] fluorodeoxyglucose and [18F] fluorocholine are two prominent examples of radiotracers that are used in diagnostics by positron emission tomography (PET). They are labeled with the radionuclide [18F] fluorine. During the radioactive decay of this unstable isotope, a proton in the nucleus of [18F] fluorine changes to a neutron. This is accompanied by the emission of a neutrino and a positron. The latter combines with an electron in the surrounding tissue, resulting in annihilation of both particles and emission of two photons (gamma rays) in opposite directions, each with an energy of 0.511 MeV. From the data acquired through coincidence detection of the photon pair, the location of its emission in the patient’s body is calculated. This location coincides closely with the location of the original radiotracer molecule, and thus reveals information on its activity.
The purity of radiotracers is of crucial importance. The highly energetic gamma rays emitted during the combination of a positron with an electron are harmful to the human body. By using a pure radiotracer, thereby avoiding the injection of free [18F] fluorine or other radioactive contaminants, the amount of radioactive substance administered to the patient can be kept to a minimum.
The quality control of radiotracers is established by radio IC in the short time between their synthesis and the recording of the three-dimensional PET scan. The separation step in radio IC is the same as in regular IC, except that it happens behind lead doors. What really sets radio IC apart from conventional IC is the detection step, in which a radioactivity detector is added to the setup. The radioactivity chromatogram reveals the presence – or, ideally, the absence – of radioactive contaminants.
Conclusion
Today, IC covers a diverse field of applications in the pharma industry (See Table 2). The technique has become extremely versatile to the user with the large number of available columns, eluent and gradient options, varied sample preparation techniques, and automation possibilities.
Table 2. Selection of IC Applications in the Pharmaceutical Industry | |||
Pharmaceutical or Excipient | Analyte | Pharmaceutical or Excipient | Analyte |
Acamprosate calcium | Acetate | Glycine carbonate, sodium salt | Carbonate |
Acifluorfen, sodium | Acetate | Glimepiride | Trans-4-methylcyclohexylamine |
Adrenaline | Adrenaline | Guaifenesin | Epichlorhydrine |
Amisulpride | Dimethyl and diethyl sulfate | Heparin sodium | Glucosamine and galactosamine |
Anticoagulation solution | Phosphate, citrate | Ibandronic acid sodium | Ibandronate, phosphite, phosphate |
Arsenic trioxide | Arsenate, arsenite | Indinavir sulfate | Ethyl sulfate |
Atovaquone | Acetate | Indomethacin sodium | 2-ethylhexane acid |
Atorvastatin calcium salt | Cyanide, tetrabutylammonium | Irbesartan | Cyanide, azide |
Sulfobutylether-ß-cyclodextrin | ß-cyclodextrin | Ibuprofen | Ibuprofen, valerophenone |
Bethanechol chloride | Bethanechol, sodium, calcium, decomposition product (HPTA) | Lamotrigine | Cyanide |
Bromide salt | Chloride | Lanthanum carbonate | Nitrate |
Busulfan | Methanesulfonic acid | Levetiracetam | Tetrabutylammonium |
Calcium gluconate | Oxalate | Levofloxacin | Fluoride |
Calcium salt | Borate | Linezolid | Morpholine |
Camphorsulfonic acid | Camphorsulfonic acid | Losartan potassium | Azide |
Carbamazepine | Chloride, bromide | Meropenem | EDTA, dimethylamine |
Carbidopa | EDTA, hydrazine, sodium disulfite | Metformin hydrochloride | Dimethylamine |
Cefadroxil | Cefadroxil | (Mono)sulfiram (temosol) | Cyanide |
Cefdinir | Iron, EDTA | Montelukast sodium | Methanesulfonic acid, acetate |
Cefepime hydrochloride | N-methyl-pyrrolidinium | Multivitamin tablets | Cations, Vitamin C |
Ceftazidime sodium | Sodium | Mycophenolate mofetil | Morpholine |
Clopidogrel besylate | Anions, carbonate, cations | Nebivolol hydrochloride | Monomethylamine |
Colesevelam | Quaternary alkylamines | Neomycin sulfate | Neomycin |
Copovidone | EP Acetate, formate | Oxaliplatin | Chloride |
Dasatinib | Ethylenediamine | Pioglitazone hydrochloride | Piperidine |
Dextromethorphan HBr | Formic acid | Piperacillin | Chloride |
(2,3-Dichlorophenyl) oxoacetonitrile | Cyanide, tetrabutylammonium | Piperazine | Piperazine, N-methylpiperazine |
Diclofenac sodium | Sodium, potassium | RA-Thermoseal toothpaste | Potassium, zinc |
Dicyclopropylmethylamine | Dicyclopropylmethylamine | Ribitol | Ribitol (adonitol) |
Doxazosin, methanesulfonic acid | Bromide | S-Adenosyl methionine | Sulfate |
Drospirenone | Propargyl alcohol | Sevelamer | Binding capacity of phosphate |
Enoxaparin sodium | Sulfate | Suxamethonium chloride | Choline chloride |
Esomeprazole magnesium | Tartrate | Tadalafil | Methanolic methylamine |
Febuxostat | Hydroxylamine | Terbinafine hydrochloride | Monomethylamine, tetrabutylammonium |
Felodipine | Silicate, sodium | Topiramate | Carbohydrates, sulfate and sulfamate |
Fenofibrate | Sodium lauryl sulfate (SLS) | Triclosan | Potassium |
Ferumoxide (contrast enhancer) | Citrate | Timolol maleate | Chlorite |
Fluorouracil (also fluoruracil) | Fluoride | Varenicline tartrate salt | Trifluormethanesulfonic acid |
Gabapentin | Chloride | Voriconazole | Camphorsulfonic acid |
Gadopentetate dimeglumine | Gadolinium | Zingisol | Potassium and zinc |
Gentamicin sulfate (see page 17) | Gentamicin | Zoledronic acid | Phosphite, phosphate |
Detection method: conductivity detection with suppression; direct conductivity detection; conductivity detection with and without suppression; amperometric detection; spectrophotometric detection
Posted by Stephanie Kappes, Alfred Steinbach, and Katinka Ruth